Nowoczesne technologie w leczeniu dysfunkcji motorycznych spowodowane urazem rdzenia kręgowego.
Słowa kluczowe:
SCI, BCI, egzoszkielety, wirtualna rzeczywistośćStreszczenie
Uraz rdzenia kręgowego (SCI) to złożony stan neurologiczny, charakteryzujący się poważnymi zaburzeniami motorycznymi, czuciowymi i autonomicznymi. SCI obejmuje dwie fazy: pierwotną i wtórną. Pierwotna faza to natychmiastowy uraz mechaniczny, natomiast wtórna obejmuje kaskadę uszkodzeń wtórnych, które mogą trwać tygodniami i pogarszać stan pacjenta. SCI prowadzi do zaburzeń kontroli motorycznej i trudności funkcjonalnych, których stopień zależy od poziomu i zakresu uszkodzenia nerwu. Co roku na świecie od 250 000 do 500 000 osób doznaje urazu rdzenia kręgowego, a liczba ta stale rośnie. SCI znacząco wpływa na jakość życia pacjentów, ograniczając ich zdolność do wykonywania codziennych czynności.
Wsparcie technologiczne, takie jak interfejsy mózg-komputer, interfejsy mózg-kręgosłup i wirtualna rzeczywistość, może znacząco poprawić skuteczność terapii rehabilitacyjnych u osób z zaburzeniami ruchu. BCI i BSI mają ogromny potencjał w przywracaniu funkcji osobom z niepełnosprawnościami, umożliwiając kontrolę nad urządzeniami zewnętrznymi, takimi jak wózki inwalidzkie, oraz przywracanie połączeń między mózgiem a rdzeniem kręgowym.
Protezy neuromotoryczne, takie jak egzoszkielety kończyn górnych i dolnych, oraz miękkie rękawice robotyczne, znacząco poprawiają jakość życia pacjentów, ułatwiając im wykonywanie codziennych czynności. Terapia VR może skutecznie łagodzić ból neuropatyczny i poprawiać funkcje motoryczne, choć wymaga dalszych badań w celu jej optymalizacji. Dzięki tym zaawansowanym technologiom, osoby z SCI mogą zyskać większą niezależność i poprawić swoją jakość życia.
SCI, BCI, egzoszkielety, wirtualna rzeczywistość
Bibliografia
Anjum, A., Yazid, M. D., Fauzi Daud, M., Idris, J., Ng, A. M. H., Selvi Naicker, A., Ismail, O. H. R., Athi Kumar, R. K., & Lokanathan, Y. (2020). Spinal Cord Injury: Pathophysiology, Multimolecular Interactions, and Underlying Recovery Mechanisms. International Journal of Molecular Sciences, 21(20), 7533. https://doi.org/10.3390/ijms21207533
O’Shea, T. M., Burda, J. E., & Sofroniew, M. V. (2017). Cell biology of spinal cord injury and repair. Journal of Clinical Investigation, 127(9), 3259–3270. https://doi.org/10.1172/jci90608
De Miguel-Rubio, A., Gallego-Aguayo, I., De Miguel-Rubio, M. D., Arias-Avila, M., Lucena-Anton, D., & Alba-Rueda, A. (2023). Effectiveness of the Combined Use of a Brain–Machine Interface System and Virtual Reality as a Therapeutic Approach in Patients with Spinal Cord Injury: A Systematic Review. Healthcare, 11(24), 3189. https://doi.org/10.3390/healthcare11243189
Bryce, T. N., Biering-Sørensen, F., Finnerup, N. B., Cardenas, D. D., Defrin, R., Lundeberg, T., Norrbrink, C., Richards, J. S., Siddall, P., Stripling, T., Treede, R.-D., Waxman, S. G., Widerström-Noga, E., Yezierski, R. P., & Dijkers, M. (2011). International Spinal Cord Injury Pain Classification: part I. Background and description. Spinal Cord, 50(6), 413–417. https://doi.org/10.1038/sc.2011.156
Hashimoto, S., Nagoshi, N., Nakamura, M., & Okano, H. (2023). Regenerative medicine strategies for chronic complete spinal cord injury. Neural Regeneration Research, 19(4), 818–824. https://doi.org/10.4103/1673-5374.382230
Khorasanizadeh, M.; Yousefifard, M.; Eskian, M.; Lu, Y.; Chalangari, M.; Harrop, J.S.; Rahimi-Movaghar, V. Neurological recovery following traumatic spinal cord injury: A systematic review and meta-analysis. J. Neurosurg. 2019, 30, 683–699.
Chen, M., Chen, Z., Xiao, X., Zhou, L., Fu, R., Jiang, X., Pang, M., & Xia, J. (2022). Corticospinal circuit neuroplasticity may involve silent synapses: implications for functional recovery facilitated by neuromodulation after spinal cord injury. IBRO Neuroscience Reports. https://doi.org/10.1016/j.ibneur.2022.08.005
Cortes, M., Elder, J., Rykman, A., Murray, L., Avedissian, M., Stampas, A., Thickbroom, G. W., Pascual-Leone, A., Krebs, H. I., Valls-Sole, J., & Edwards, D. J. (2013). Improved motor performance in chronic spinal cord injury following upper-limb robotic training. NeuroRehabilitation, 33(1), 57–65. https://doi.org/10.3233/nre-130928
Wang, D.-F., Xu, D.-S., Li, Z.-Y., Huo, C.-C., Zheng, Y., Lu, W.-W., & Zhang, T.-Y. (2021). Prospects for intelligent rehabilitation techniques to treat motor dysfunction. Neural Regeneration Research, 16(2), 264. https://doi.org/10.4103/1673-5374.290884
Cappello, L., Meyer, J. T., Galloway, K. C., Peisner, J. D., Granberry, R., Wagner, D. A., Engelhardt, S., Paganoni, S., & Walsh, C. J. (2018). Assisting hand function after spinal cord injury with a fabric-based soft robotic glove. Journal of NeuroEngineering and Rehabilitation, 15(1). https://doi.org/10.1186/s12984-018-0391-x
Collinger, J. L., Boninger, M. L., Bruns, T. M., Curley, K., Wang, W., & Weber, D. J. (2013). Functional priorities, assistive technology, and brain-computer interfaces after spinal cord injury. The Journal of Rehabilitation Research and Development, 50(2), 145. https://doi.org/10.1682/jrrd.2011.11.0213
Tayebi, H., Azadnajafabad, S., Maroufi, S. F., Pour-Rashidi, A., Khorasanizadeh, M., Faramarzi, S., & Slavin, K. V. (2023). Applications of brain-computer interfaces in neurodegenerative diseases. Neurosurgical Review, 46(1). https://doi.org/10.1007/s10143-023-02038-9
Birbaumer, N., Ghanayim, N., Hinterberger, T., Iversen, I., Kotchoubey, B., Kübler, A., Perelmouter, J., Taub, E., & Flor, H. (1999). A spelling device for the paralysed. Nature, 398(6725), 297–298. https://doi.org/10.1038/18581
Moore, P. J., Lyons, T. J., & Gallacher, J. (2019). Using path signatures to predict a diagnosis of Alzheimer’s disease. PLOS ONE, 14(9), Artykuł e0222212. https://doi.org/10.1371/journal.pone.0222212
Rupp, R. (2014). Challenges in clinical applications of brain computer interfaces in individuals with spinal cord injury. Frontiers in Neuroengineering, 7. https://doi.org/10.3389/fneng.2014.00038
Akhtari, M., Bryant, H. C., Mamelak, A. N. M., Heller, L., Shih, J. J., Mandelkern, M., Matlachov, A., Ranken, D. M., Best, E. D., & Sutherling, W. W. (2000). Brain Topography, 13(1), 29–42. https://doi.org/10.1023/a:1007882102297
Strait, M., & Scheutz, M. (2014). What we can and cannot (yet) do with functional near infrared spectroscopy. Frontiers in Neuroscience, 8. https://doi.org/10.3389/fnins.2014.00117
Pfurtscheller, G. (2010). The hybrid BCI. Frontiers in Neuroscience. https://doi.org/10.3389/fnpro.2010.00003
Waldert, S., Pistohl, T., Braun, C., Ball, T., Aertsen, A., & Mehring, C. (2009). A review on directional information in neural signals for brain-machine interfaces. Journal of Physiology-Paris, 103(3-5), 244–254. https://doi.org/10.1016/j.jphysparis.2009.08.007
Levett, J. J., Elkaim, L. M., Niazi, F., Weber, M. H., Iorio-Morin, C., Bonizzato, M., & Weil, A. G. (2023). Invasive Brain Computer Interface for Motor Restoration in Spinal Cord Injury: A Systematic Review. Neuromodulation: Technology at the Neural Interface. https://doi.org/10.1016/j.neurom.2023.10.006 Birbaumer, N. (2006). Brain–computer-interface research: Coming of age. Clinical Neurophysiology, 117(3), 479–483. https://doi.org/10.1016/j.clinph.2005.11.002
Li, F., Chen, B., Li, H., Zhang, T., Wang, F., Jiang, Y., Li, P., Ma, T., Zhang, R., Tian, Y., Liu, T., Guo, D., Yao, D., & Xu, P. (2016a). The Time-Varying Networks in P300:_newline A Task-Evoked EEG Study. IEEE Transactions on Neural Systems and Rehabilitation Engineering, 24(7), 725–733. https://doi.org/10.1109/tnsre.2016.2523678
Chen, X., Yu, Y., Tang, J., Zhou, L., Liu, K., Liu, Z., Chen, S., Wang, J., Zeng, L.-L., Liu, J., & Hu, D. (2022). Clinical Validation of BCI-Controlled Wheelchairs in Subjects With Severe Spinal Cord Injury. IEEE Transactions on Neural Systems and Rehabilitation Engineering, 30, 579–589. https://doi.org/10.1109/tnsre.2022.3156661
Erickson, M. L., Ryan, T. E., Young, H.-J., & McCully, K. K. (2013). Near-infrared assessments of skeletal muscle oxidative capacity in persons with spinal cord injury. European Journal of Applied Physiology, 113(9), 2275–2283. https://doi.org/10.1007/s00421-013-2657-0
Ghatas, M. P., Holman, M. E., & Gorgey, A. S. (2019). Methodological considerations for near-infrared spectroscopy to assess mitochondrial capacity after spinal cord injury. The Journal of Spinal Cord Medicine, 43(5), 623–632. https://doi.org/10.1080/10790268.2019.1631585
Rashnavadi, T., Macnab, A., Cheung, A., Shadgan, A., Kwon, B. K., & Shadgan, B. (2019). Monitoring spinal cord hemodynamics and tissue oxygenation: a review of the literature with special focus on the near-infrared spectroscopy technique. Spinal Cord, 57(8), 617–625. https://doi.org/10.1038/s41393-019-0304-2
Cajigas, I., Davis, K. C., Prins, N. W., Gallo, S., Naeem, J. A., Fisher, L., Ivan, M. E., Prasad, A., & Jagid, J. R. (2023). Brain-Computer interface control of stepping from invasive electrocorticography upper-limb motor imagery in a patient with quadriplegia. Frontiers in Human Neuroscience, 16. https://doi.org/10.3389/fnhum.2022.1077416
Wang, W., Collinger, J. L., Degenhart, A. D., Tyler-Kabara, E. C., Schwartz, A. B., Moran, D. W., Weber, D. J., Wodlinger, B., Vinjamuri, R. K., Ashmore, R. C., Kelly, J. W., & Boninger, M. L. (2013). An Electrocorticographic Brain Interface in an Individual with Tetraplegia. PLoS ONE, 8(2), Artykuł e55344. https://doi.org/10.1371/journal.pone.0055344
Huggins, J. E., Moinuddin, A. A., Chiodo, A. E., & Wren, P. A. (2015). What Would Brain-Computer Interface Users Want: Opinions and Priorities of Potential Users With Spinal Cord Injury. Archives of Physical Medicine and Rehabilitation, 96(3), S38—S45.e5. https://doi.org/10.1016/j.apmr.2014.05.028
Davis, K. C., Meschede-Krasa, B., Cajigas, I., Prins, N. W., Alver, C., Gallo, S., Bhatia, S., Abel, J. H., Naeem, J. A., Fisher, L., Raza, F., Rifai, W. R., Morrison, M., Ivan, M. E., Brown, E. N., Jagid, J. R., & Prasad, A. (2022). Design-development of an at-home modular brain–computer interface (BCI) platform in a case study of cervical spinal cord injury. Journal of NeuroEngineering and Rehabilitation, 19(1). https://doi.org/10.1186/s12984-022-01026-2
Romanelli, P., Piangerelli, M., Ratel, D., Gaude, C., Costecalde, T., Puttilli, C., Picciafuoco, M., Benabid, A., & Torres, N. (2019). A novel neural prosthesis providing long-term electrocorticography recording and cortical stimulation for epilepsy and brain-computer interface. Journal of Neurosurgery, 130(4), 1166–1179. https://doi.org/10.3171/2017.10.jns17400
Zulauf-Czaja, A., Al-Taleb, M. K. H., Purcell, M., Petric-Gray, N., Cloughley, J., & Vuckovic, A. (2021). On the way home: a BCI-FES hand therapy self-managed by sub-acute SCI participants and their caregivers: a usability study. Journal of NeuroEngineering and Rehabilitation, 18(1). https://doi.org/10.1186/s12984-021-00838-y
Wenger, N., Moraud, E. M., Raspopovic, S., Bonizzato, M., DiGiovanna, J., Musienko, P., Morari, M., Micera, S., & Courtine, G. (2014). Closed-loop neuromodulation of spinal sensorimotor circuits controls refined locomotion after complete spinal cord injury. Science Translational Medicine, 6(255), 255ra133. https://doi.org/10.1126/scitranslmed.3008325
Hersh, A. M., Weber-Levine, C., Jiang, K., & Theodore, N. (2023). Spinal Cord Injury. Neurosurgery Clinics of North America. https://doi.org/10.1016/j.nec.2023.10.001
Danner, S. M., Hofstoetter, U. S., Freundl, B., Binder, H., Mayr, W., Rattay, F., & Minassian, K. (2015). Human spinal locomotor control is based on flexibly organized burst generators. Brain, 138(3), 577–588. https://doi.org/10.1093/brain/awu372
Capogrosso, M., Milekovic, T., Borton, D., Wagner, F., Moraud, E. M., Mignardot, J.-B., Buse, N., Gandar, J., Barraud, Q., Xing, D., Rey, E., Duis, S., Jianzhong, Y., Ko, W. K. D., Li, Q., Detemple, P., Denison, T., Micera, S., Bezard, E., ... Courtine, G. (2016). A brain–spine interface alleviating gait deficits after spinal cord injury in primates. Nature, 539(7628), 284–288. https://doi.org/10.1038/nature20118
Lorach, H., Galvez, A., Spagnolo, V., Martel, F., Karakas, S., Intering, N., Vat, M., Faivre, O., Harte, C., Komi, S., Ravier, J., Collin, T., Coquoz, L., Sakr, I., Baaklini, E., Hernandez-Charpak, S. D., Dumont, G., Buschman, R., Buse, N., ... Courtine, G. (2023). Walking naturally after spinal cord injury using a brain–spine interface. Nature. https://doi.org/10.1038/s41586-023-06094-5
Lorach, H., Charvet, G., Bloch, J., & Courtine, G. (2022). Brain-spine interfaces to reverse paralysis. National Science Review. https://doi.org/10.1093/nsr/nwac009
Wagner, F. B., Mignardot, J.-B., Le Goff-Mignardot, C. G., Demesmaeker, R., Komi, S., Capogrosso, M., Rowald, A., Seáñez, I., Caban, M., Pirondini, E., Vat, M., McCracken, L. A., Heimgartner, R., Fodor, I., Watrin, A., Seguin, P., Paoles, E., Van Den Keybus, K., Eberle, G., ... Courtine, G. (2018). Targeted neurotechnology restores walking in humans with spinal cord injury. Nature, 563(7729), 65–71. https://doi.org/10.1038/s41586-018-0649-2
Benabid, A. L., Costecalde, T., Eliseyev, A., Charvet, G., Verney, A., Karakas, S., Foerster, M., Lambert, A., Morinière, B., Abroug, N., Schaeffer, M.-C., Moly, A., Sauter-Starace, F., Ratel, D., Moro, C., Torres-Martinez, N., Langar, L., Oddoux, M., Polosan, M., ... Chabardes, S. (2019). An exoskeleton controlled by an epidural wireless brain–machine interface in a tetraplegic patient: a proof-of-concept demonstration. The Lancet Neurology, 18(12), 1112–1122. https://doi.org/10.1016/s1474-4422(19)30321-7
Hochberg, L. R., Serruya, M. D., Friehs, G. M., Mukand, J. A., Saleh, M., Caplan, A. H., Branner, A., Chen, D., Penn, R. D., & Donoghue, J. P. (2006). Neuronal ensemble control of prosthetic devices by a human with tetraplegia. Nature, 442(7099), 164–171. https://doi.org/10.1038/nature04970
Chouhan, A., Trikha, S., Dhawle, S., Nagwanshi, J., & Chandra, S. (2014). DOI: 10.14260/jemds/201 5 / 1 0 ORIGINAL ARTICLE J of Evolution of Med and Dent Sci/ eISSN - 2278 - 4802 , pISSN - 2278 - 4748/ Vol . 4 / Issue 01 / Jan 01 , 201 5 Page 59 A STUDY OF CORRELATION OF ESOPHAGEAL VARICES IN CIRRHOTIC PATIENTS WITH PORTAL HAEMODYNAMICS WITH SPECIAL REFERENCE TO PORTAL VEIN DIAMETER, PORTAL VEIN VELOCITY, CONGESTION INDEX, LIVER VASCULAR INDEX. Journal of Evolution of Medical and Dental Sciences, 4(01), 59–66. https://doi.org/10.14260/jemds/2015/10
Cortes, M., Elder, J., Rykman, A., Murray, L., Avedissian, M., Stampas, A., Thickbroom, G. W., Pascual-Leone, A., Krebs, H. I., Valls-Sole, J., & Edwards, D. J. (2013). Improved motor performance in chronic spinal cord injury following upper-limb robotic training. NeuroRehabilitation, 33(1), 57–65. https://doi.org/10.3233/nre-130928
Pehlivan, A. U., Sergi, F., Erwin, A., Yozbatiran, N., Francisco, G. E., & O'Malley, M. K. (2014). Design and validation of the RiceWrist-S exoskeleton for robotic rehabilitation after incomplete spinal cord injury. Robotica, 32(8), 1415–1431. https://doi.org/10.1017/s0263574714001490
Lau, J. C. L., & Mombaur, K. (2022). Preliminary Study on a Novel Protocol for Improving Familiarity with a Lower-Limb Robotic Exoskeleton in Able-Bodied, First-Time Users. Frontiers in Robotics and AI, 8. https://doi.org/10.3389/frobt.2021.785251
Mekki, M., Delgado, A. D., Fry, A., Putrino, D., & Huang, V. (2018). Robotic Rehabilitation and Spinal Cord Injury: a Narrative Review. Neurotherapeutics, 15(3), 604–617. https://doi.org/10.1007/s13311-018-0642-3
Tefertiller, C., Hays, K., Jones, J., Jayaraman, A., Hartigan, C., Bushnik, T., & Forrest, G. F. (2018). Initial Outcomes from a Multicenter Study Utilizing the Indego Powered Exoskeleton in Spinal Cord Injury. Topics in Spinal Cord Injury Rehabilitation, 24(1), 78–85. https://doi.org/10.1310/sci17-00014
Contreras-Vidal, J. L., A Bhagat, N., Brantley, J., Cruz-Garza, J. G., He, Y., Manley, Q., Nakagome, S., Nathan, K., Tan, S. H., Zhu, F., & Pons, J. L. (2016). Powered exoskeletons for bipedal locomotion after spinal cord injury. Journal of Neural Engineering, 13(3), 031001. https://doi.org/10.1088/1741-2560/13/3/031001
Yurkewich, A., Kozak, I. J., Ivanovic, A., Rossos, D., Wang, R. H., Hebert, D., & Mihailidis, A. (2020). Myoelectric untethered robotic glove enhances hand function and performance on daily living tasks after stroke. Journal of Rehabilitation and Assistive Technologies Engineering, 7, 205566832096405. https://doi.org/10.1177/2055668320964050
Yurkewich, A., Ortega, S., Sanchez, J., Wang, R. H., & Burdet, E. (2022). Integrating hand exoskeletons into goal-oriented clinic and home stroke and spinal cord injury rehabilitation. Journal of Rehabilitation and Assistive Technologies Engineering, 9, 205566832211309. https://doi.org/10.1177/20556683221130970
Mekki, M., Delgado, A. D., Fry, A., Putrino, D., & Huang, V. (2018). Robotic Rehabilitation and Spinal Cord Injury: a Narrative Review. Neurotherapeutics, 15(3), 604–617. https://doi.org/10.1007/s13311-018-0642-3
Rudhe, C., Albisser, U., Starkey, M. L., Curt, A., & Bolliger, M. (2012). Reliability of movement workspace measurements in a passive arm orthosis used in spinal cord injury rehabilitation. Journal of NeuroEngineering and Rehabilitation, 9(1), 37. https://doi.org/10.1186/1743-0003-9-37
Zariffa, J., Kapadia, N., Kramer, J. L. K., Taylor, P., Alizadeh-Meghrazi, M., Zivanovic, V., Albisser, U., Willms, R., Townson, A., Curt, A., Popovic, M. R., & Steeves, J. D. (2012). Relationship Between Clinical Assessments of Function and Measurements From an Upper-Limb Robotic Rehabilitation Device in Cervical Spinal Cord Injury. IEEE Transactions on Neural Systems and Rehabilitation Engineering, 20(3), 341–350. https://doi.org/10.1109/tnsre.2011.2181537
Cortes, M., Elder, J., Rykman, A., Murray, L., Avedissian, M., Stampas, A., Thickbroom, G. W., Pascual-Leone, A., Krebs, H. I., Valls-Sole, J., & Edwards, D. J. (2013). Improved motor performance in chronic spinal cord injury following upper-limb robotic training. NeuroRehabilitation, 33(1), 57–65. https://doi.org/10.3233/nre-130928
Susanto, E. A., Tong, R. K., Ockenfeld, C., & Ho, N. S. (2015). Efficacy of robot-assisted fingers training in chronic stroke survivors: a pilot randomized-controlled trial. Journal of NeuroEngineering and Rehabilitation, 12(1). https://doi.org/10.1186/s12984-015-0033-5
Cappello, L., Meyer, J. T., Galloway, K. C., Peisner, J. D., Granberry, R., Wagner, D. A., Engelhardt, S., Paganoni, S., & Walsh, C. J. (2018). Assisting hand function after spinal cord injury with a fabric-based soft robotic glove. Journal of NeuroEngineering and Rehabilitation, 15(1). https://doi.org/10.1186/s12984-018-0391-x
Soekadar, S. R., Witkowski, M., Gómez, C., Opisso, E., Medina, J., Cortese, M., Cempini, M., Carrozza, M. C., Cohen, L. G., Birbaumer, N., & Vitiello, N. (2016). Hybrid EEG/EOG-based brain/neural hand exoskeleton restores fully independent daily living activities after quadriplegia. Science Robotics, 1(1), Artykuł eaag3296. https://doi.org/10.1126/scirobotics.aag3296
Miguel-Rubio, A. D., Rubio, M. D., Salazar, A., Moral-Munoz, J. A., Requena, F., Camacho, R., & Lucena-Anton, D. (2020). Is Virtual Reality Effective for Balance Recovery in Patients with Spinal Cord Injury? A Systematic Review and Meta-Analysis. Journal of Clinical Medicine, 9(9), 2861. https://doi.org/10.3390/jcm9092861
Chi, B., Chau, B., Yeo, E., & Ta, P. (2019). Virtual reality for spinal cord injury-associated neuropathic pain: Systematic review. Annals of Physical and Rehabilitation Medicine, 62(1), 49–57. https://doi.org/10.1016/j.rehab.2018.09.006
Jensen, T. S., Baron, R., Haanpää, M., Kalso, E., Loeser, J. D., Rice, A. S. C., & Treede, R.-D. (2011). A new definition of neuropathic pain. Pain, 152(10), 2204–2205. https://doi.org/10.1016/j.pain.2011.06.017
Burke, D., Fullen, B. M., Stokes, D., & Lennon, O. (2016). Neuropathic pain prevalence following spinal cord injury: A systematic review and meta-analysis. European Journal of Pain, 21(1), 29–44. https://doi.org/10.1002/ejp.905
Kumru, H., Soler, D., Vidal, J., Navarro, X., Tormos, J. M., Pascual-Leone, A., & Valls-Sole, J. (2012). The effects of transcranial direct current stimulation with visual illusion in neuropathic pain due to spinal cord injury: An evoked potentials and quantitative thermal testing study. European Journal of Pain, 17(1), 55–66. https://doi.org/10.1002/j.1532-2149.2012.00167.x
Soler, M. D., Kumru, H., Pelayo, R., Vidal, J., Tormos, J. M., Fregni, F., Navarro, X., & Pascual-Leone, A. (2010). Effectiveness of transcranial direct current stimulation and visual illusion on neuropathic pain in spinal cord injury. Brain, 133(9), 2565–2577. https://doi.org/10.1093/brain/awq184
Villiger, M., Bohli, D., Kiper, D., Pyk, P., Spillmann, J., Meilick, B., Curt, A., Hepp-Reymond, M.-C., Hotz-Boendermaker, S., & Eng, K. (2013). Virtual Reality–Augmented Neurorehabilitation Improves Motor Function and Reduces Neuropathic Pain in Patients With Incomplete Spinal Cord Injury. Neurorehabilitation and Neural Repair, 27(8), 675–683. https://doi.org/10.1177/1545968313490999
Pozeg, P., Palluel, E., Ronchi, R., Solcà, M., Al-Khodairy, A.-W., Jordan, X., Kassouha, A., & Blanke, O. (2017). Virtual reality improves embodiment and neuropathic pain caused by spinal cord injury. Neurology, 89(18), 1894–1903. https://doi.org/10.1212/wnl.0000000000004585
Oneal, B. J., Patterson, D. R., Soltani, M., Teeley, A., & Jensen, M. P. (2008). Virtual Reality Hypnosis in the Treatment of Chronic Neuropathic Pain:A Case Report. International Journal of Clinical and Experimental Hypnosis, 56(4), 451–462. https://doi.org/10.1080/00207140802255534
Miguel-Rubio, A. D., Rubio, M. D., Salazar, A., Moral-Munoz, J. A., Requena, F., Camacho, R., & Lucena-Anton, D. (2020b). Is Virtual Reality Effective for Balance Recovery in Patients with Spinal Cord Injury? A Systematic Review and Meta-Analysis. Journal of Clinical Medicine, 9(9), 2861. https://doi.org/10.3390/jcm9092861
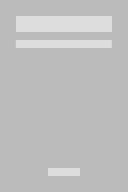
Opublikowane
Licencja

Utwór dostępny jest na licencji Creative Commons Uznanie autorstwa – Użycie niekomercyjne – Bez utworów zależnych 4.0 Międzynarodowe.