Współczesne perspektywy wykorzystania microRNA w terapii i diagnostyce glejaka wielopostaciowego
Słowa kluczowe:
glejak wielopostaciowy, miRNA, epigenetyka, diagnostyka, terapiaStreszczenie
Glejak wielopostaciowy [ICD-10:C71.9] zaliczany do guzów złośliwych mózgu IV stopnia, jest jednym z najgorzej rokujących nowotworów tego narządu, z medianą przeżycia na poziomie 12-18 miesięcy. Obecnie stosowanie metody terapeutyczne z wykorzystaniem leczenia operacyjnego, radioterapii czy chemioterapii, balansują na cienkiej granicy efektownego zniszczenia nowotworu przy minimalizacji jatrogennych błędów, mogących skutkować deficytami neurologicznymi. Problematyka postępowania dotyczy także samej diagnostyki, gdzie rozpoznanie guza na podstawie obrazu rezonansu magnetycznego nie pozwala na jego klasyfikację niezbędną do doboru odpowiedniej terapii, w związku z czym konieczne jest wykorzystanie inwazyjnych metod diagnostycznych. Stwarza to wyraźną potrzebę znalezienia nowych możliwości postępowania w glejaku wielopostaciowym, gdzie obiecującą perspektywę stwarzają cząsteczki miRNA. Są to jednoniciowe, małe, niekodujące cząsteczki RNA, odpowiedzialne za wyciszenie potranskrypcyjne ekspresji genów, regulujących m.in.: procesy replikacji komórkowej, apoptozy, angiogenezy czy naprawy DNA. W ostatnich latach udowodniono, że w komórkach glejaka wielopostaciowego dochodzi do zmian ekspresji i stężenia prawie 350 cząsteczek miRNA, co może świadczyć o ich kluczowym znaczeniu w epigenetycznych mechanizmach patogenezy tego nowotworu. Korelacja ich stężenia z nasileniem kancerogenezy, otwiera drogę do wykorzystania ich jako markerów nowotworowych i stworzenia nowych niekonwencjonalnych metod diagnostycznych. Ponadto możliwość inhibicji lub substytucji odpowiednich cząsteczek miRNA stanowi zupełnie nowe podejście terapeutyczne, dające obiecujące wyniki w próbach na modelach zwierzęcych. Jednakże nie zbadano jeszcze dokładnej sygnatury miRNA dla glejaka wielopostaciowego, wiele cząsteczek ma nieznane wielokierunkowe działanie a ich ilość w komórkach przekracza liczbę 3000, dlatego konieczne są dalsze badania pozwalające na dokładne określenie ich funkcji w komórce i znalezienie możliwych sposobów ich wykorzystania w praktyce klinicznej.
Bibliografia
Md. S. Uddin et al., “Epigenetics of glioblastoma multiforme: From molecular mechanisms to therapeutic approaches,” Semin Cancer Biol, vol. 83, pp. 100–120, Aug. 2022, doi: 10.1016/j.semcancer.2020.12.015.
A. O. Sasmita, Y. P. Wong, and A. P. K. Ling, “Biomarkers and therapeutic advances in glioblastoma multiforme,” Asia Pac J Clin Oncol, vol. 14, no. 1, pp. 40–51, Feb. 2018, doi: 10.1111/ajco.12756.
M. Niyazi et al., “MiRNA expression patterns predict survival in glioblastoma,” Radiation Oncology, vol. 6, no. 1, p. 153, Dec. 2011, doi: 10.1186/1748-717X-6-153.
S. P. Ahmed, J. S. Castresana, and M. H. Shahi, “Glioblastoma and MiRNAs,” Cancers (Basel), vol. 13, no. 7, p. 1581, Mar. 2021, doi: 10.3390/cancers13071581.
F. H. Pottoo, Md. N. Javed, J. U. Rahman, T. Abu-Izneid, and F. A. Khan, “Targeted delivery of miRNA based therapeuticals in the clinical management of Glioblastoma Multiforme,” Semin Cancer Biol, vol. 69, pp. 391–398, Feb. 2021, doi: 10.1016/j.semcancer.2020.04.001.
H. Wang, R. Peng, J. Wang, Z. Qin, and L. Xue, “Circulating microRNAs as potential cancer biomarkers: the advantage and disadvantage,” Clin Epigenetics, vol. 10, no. 1, p. 59, Dec. 2018, doi: 10.1186/s13148-018-0492-1.
B. K. Ahir, H. Ozer, H. H. Engelhard, and S. S. Lakka, “MicroRNAs in glioblastoma pathogenesis and therapy: A comprehensive review,” Crit Rev Oncol Hematol, vol. 120, pp. 22–33, Dec. 2017, doi: 10.1016/j.critrevonc.2017.10.003.
I. S. E. E. Sati and I. Parhar, “MicroRNAs Regulate Cell Cycle and Cell Death Pathways in Glioblastoma,” Int J Mol Sci, vol. 22, no. 24, p. 13550, Dec. 2021, doi: 10.3390/ijms222413550.
F. Hanif, K. Muzaffar, K. Perveen, S. M. Malhi, and S. U. Simjee, “Glioblastoma Multiforme: A Review of its Epidemiology and Pathogenesis through Clinical Presentation and Treatment.,” Asian Pac J Cancer Prev, vol. 18, no. 1, pp. 3–9, Jan. 2017, doi: 10.22034/APJCP.2017.18.1.3.
E. Bigos, M. Spych, M. Masłowski, L. Gottwald, and J. Fijuth, “Glejak wielopostaciowy mózgu - problem coraz bardziej aktualny,” Medycyna Paliatywna, vol. 6, no. 1, pp. 7–13, 2014.
M.-T. Bahreyni-Toossi, E. Dolat, H. Khanbabaei, N. Zafari, and H. Azimian, “microRNAs: Potential glioblastoma radiosensitizer by targeting radiation-related molecular pathways,” Mutation Research/Fundamental and Molecular Mechanisms of Mutagenesis, vol. 816–818, p. 111679, Nov. 2019, doi: 10.1016/j.mrfmmm.2019.111679.
C. Alifieris and D. T. Trafalis, “Glioblastoma multiforme: Pathogenesis and treatment,” Pharmacol Ther, vol. 152, pp. 63–82, Aug. 2015, doi: 10.1016/j.pharmthera.2015.05.005.
S. Grochans et al., “Epidemiology of Glioblastoma Multiforme–Literature Review,” Cancers (Basel), vol. 14, no. 10, p. 2412, May 2022, doi: 10.3390/cancers14102412.
D. Simińska et al., “Epidemiology of Anthropometric Factors in Glioblastoma Multiforme—Literature Review,” Brain Sci, vol. 11, no. 1, p. 116, Jan. 2021, doi: 10.3390/brainsci11010116.
R. Salami, M. Salami, A. Mafi, O. Vakili, and Z. Asemi, “Circular RNAs and glioblastoma multiforme: focus on molecular mechanisms,” Cell Communication and Signaling, vol. 20, no. 1, p. 13, Jan. 2022, doi: 10.1186/s12964-021-00809-9.
R. G. W. Verhaak et al., “Integrated Genomic Analysis Identifies Clinically Relevant Subtypes of Glioblastoma Characterized by Abnormalities in PDGFRA, IDH1, EGFR, and NF1,” Cancer Cell, vol. 17, no. 1, pp. 98–110, Jan. 2010, doi: 10.1016/j.ccr.2009.12.020.
G. S. Stoyanov, D. Dzhenkov, P. Ghenev, B. Iliev, Y. Enchev, and A. B. Tonchev, “Cell biology of glioblastoma multiforme: from basic science to diagnosis and treatment,” Medical Oncology, vol. 35, no. 3, p. 27, Mar. 2018, doi: 10.1007/s12032-018-1083-x.
Z. Dong and H. Cui, “Epigenetic modulation of metabolism in glioblastoma,” Semin Cancer Biol, vol. 57, pp. 45–51, Aug. 2019, doi: 10.1016/j.semcancer.2018.09.002.
V. Simion et al., “Intracellular trafficking and functional monitoring of miRNA delivery in glioblastoma using lipopolyplexes and the miRNA-ON RILES reporter system,” Journal of Controlled Release, vol. 327, pp. 429–443, Nov. 2020, doi: 10.1016/j.jconrel.2020.08.028.
O. Rezaei, K. Honarmand, S. Nateghinia, M. Taheri, and S. Ghafouri-Fard, “miRNA signature in glioblastoma: Potential biomarkers and therapeutic targets,” Exp Mol Pathol, vol. 117, p. 104550, Dec. 2020, doi: 10.1016/j.yexmp.2020.104550.
J. W. Luo, X. Wang, Y. Yang, and Q. Mao, “Role of micro-RNA (miRNA) in pathogenesis of glioblastoma.,” Eur Rev Med Pharmacol Sci, vol. 19, no. 9, pp. 1630–9, 2015.
J. A. Chan, A. M. Krichevsky, and K. S. Kosik, “MicroRNA-21 Is an Antiapoptotic Factor in Human Glioblastoma Cells,” Cancer Res, vol. 65, no. 14, pp. 6029–6033, Jul. 2005, doi: 10.1158/0008-5472.CAN-05-0137.
A. Shea et al., “MicroRNAs in glioblastoma multiforme pathogenesis and therapeutics,” Cancer Med, vol. 5, no. 8, pp. 1917–1946, Aug. 2016, doi: 10.1002/cam4.775.
A. Buruiană et al., “The Roles of miRNA in Glioblastoma Tumor Cell Communication: Diplomatic and Aggressive Negotiations,” Int J Mol Sci, vol. 21, no. 6, p. 1950, Mar. 2020, doi: 10.3390/ijms21061950.
L. Chen and C. Kang, “miRNA interventions serve as ‘magic bullets’ in the reversal of glioblastoma hallmarks,” Oncotarget, vol. 6, no. 36, pp. 38628–38642, Nov. 2015, doi: 10.18632/oncotarget.5926.
F. Talotta et al., “An autoregulatory loop mediated by miR-21 and PDCD4 controls the AP-1 activity in RAS transformation,” Oncogene, vol. 28, no. 1, pp. 73–84, Jan. 2009, doi: 10.1038/onc.2008.370.
S.-T. Lee et al., “Let-7 microRNA inhibits the proliferation of human glioblastoma cells,” J Neurooncol, vol. 102, no. 1, pp. 19–24, Mar. 2011, doi: 10.1007/s11060-010-0286-6.
Y. Liu et al., “microRNA-200a downregulation in human glioma leads to Gαi1 over-expression, Akt activation, and cell proliferation,” Oncogene, vol. 37, no. 21, pp. 2890–2902, May 2018, doi: 10.1038/s41388-018-0184-5.
Y. Li et al., “MicroRNA-34a Inhibits Glioblastoma Growth by Targeting Multiple Oncogenes,” Cancer Res, vol. 69, no. 19, pp. 7569–7576, Oct. 2009, doi: 10.1158/0008-5472.CAN-09-0529.
T. Papagiannakopoulos, A. Shapiro, and K. S. Kosik, “MicroRNA-21 Targets a Network of Key Tumor-Suppressive Pathways in Glioblastoma Cells,” Cancer Res, vol. 68, no. 19, pp. 8164–8172, Oct. 2008, doi: 10.1158/0008-5472.CAN-08-1305.
C.-Z. Zhang et al., “MiR-221 and miR-222 target PUMA to induce cell survival in glioblastoma,” Mol Cancer, vol. 9, no. 1, p. 229, Dec. 2010, doi: 10.1186/1476-4598-9-229.
H. Xia et al., “MiR-218 sensitizes glioma cells to apoptosis and inhibits tumorigenicity by regulating ECOP-mediated suppression of NF-κB activity,” Neuro Oncol, vol. 15, no. 4, pp. 413–422, Apr. 2013, doi: 10.1093/neuonc/nos296.
Y. Nan et al., “MiRNA-451 plays a role as tumor suppressor in human glioma cells,” Brain Res, vol. 1359, pp. 14–21, Nov. 2010, doi: 10.1016/j.brainres.2010.08.074.
M. H. Pourhanifeh et al., “Autophagy in cancers including brain tumors: role of MicroRNAs,” Cell Communication and Signaling, vol. 18, no. 1, p. 88, Dec. 2020, doi: 10.1186/s12964-020-00587-w.
S. Comincini et al., “microRNA-17 regulates the expression of ATG7 and modulates the autophagy process, improving the sensitivity to temozolomide and low-dose ionizing radiation treatments in human glioblastoma cells,” Cancer Biol Ther, vol. 14, no. 7, pp. 574–586, Jul. 2013, doi: 10.4161/cbt.24597.
H.-S. Gwak et al., “Silencing of MicroRNA-21 Confers Radio-Sensitivity through Inhibition of the PI3K/AKT Pathway and Enhancing Autophagy in Malignant Glioma Cell Lines,” PLoS One, vol. 7, no. 10, p. e47449, Oct. 2012, doi: 10.1371/journal.pone.0047449.
P. Guo et al., “MiR-26a enhances the radiosensitivity of glioblastoma multiforme cells through targeting of ataxia–telangiectasia mutated,” Exp Cell Res, vol. 320, no. 2, pp. 200–208, Jan. 2014, doi: 10.1016/j.yexcr.2013.10.020.
H. I. Yoon et al., “The Korean Society for Neuro-Oncology (KSNO) Guideline for Adult Diffuse Midline Glioma: Version 2021.1,” Brain Tumor Res Treat, vol. 9, no. 1, p. 1, 2021, doi: 10.14791/btrt.2021.9.e8.
B. M. Ellingson, P. Y. Wen, and T. F. Cloughesy, “Modified Criteria for Radiographic Response Assessment in Glioblastoma Clinical Trials,” Neurotherapeutics, vol. 14, no. 2, pp. 307–320, 2017, doi: 10.1007/s13311-016-0507-6.
E. Bigos, M. K. Spych, M. Masłowski, L. M. Gottwald, and J. Fijuth, “Glejak wielopostaciowy mózgu-problem coraz bardziej aktualny Glioblastoma-the topical problem,” Medycyna Paliatywna, vol. 6, no. 1, pp. 7–13, 2014.
N. L. Albert et al., “Response Assessment in Neuro-Oncology working group and European Association for Neuro-Oncology recommendations for the clinical use of PET imaging in gliomas,” Neuro Oncol, vol. 18, no. 9, pp. 1199–1208, 2016, doi: 10.1093/neuonc/now058.
E. Le Rhun, J. Seoane, M. Salzet, R. Soffietti, and M. Weller, “Liquid biopsies for diagnosing and monitoring primary tumors of the central nervous system,” Cancer Lett, vol. 480, no. March, pp. 24–28, 2020, doi: 10.1016/j.canlet.2020.03.021.
M. Weller et al., “EANO guideline for the diagnosis and treatment of anaplastic gliomas and glioblastoma,” Lancet Oncol, vol. 15, no. 9, pp. 395–403, 2014, doi: 10.1016/S1470-2045(14)70011-7.
C. A. Hamisch et al., “Frame-based stereotactic biopsy of deep-seated and midline structures in 511 procedures: feasibility, risk profile, and diagnostic yield,” Acta Neurochir (Wien), vol. 161, no. 10, pp. 2065–2071, 2019, doi: 10.1007/s00701-019-04020-1.
Q. T. Ostrom et al., “CBTRUS Statistical Report: Primary Brain and Other Central Nervous System Tumors Diagnosed in the United States in 2012-2016,” Neuro Oncol, vol. 21, pp. V1–V100, 2019, doi: 10.1093/neuonc/noz150.
W. Stummer, U. Pichlmeier, T. Meinel, O. D. Wiestler, F. Zanella, and H. J. Reulen, “Fluorescence-guided surgery with 5-aminolevulinic acid for resection of malignant glioma: a randomised controlled multicentre phase III trial,” Lancet Oncology, vol. 7, no. 5, pp. 392–401, 2006, doi: 10.1016/S1470-2045(06)70665-9.
J. Brennum, C. M. Maier, K. Almdal, C. M. Engelmann, and M. Gjerris, “Primo non nocere or maximum survival in grade 2 gliomas? A medical ethical question,” Acta Neurochir (Wien), vol. 157, no. 2, pp. 155–164, 2015, doi: 10.1007/s00701-014-2304-5.
S. Bette et al., “Patterns and Time Dependence of Unspecific Enhancement in Postoperative Magnetic Resonance Imaging after Glioblastoma Resection,” World Neurosurg, vol. 90, pp. 440–447, 2016, doi: 10.1016/j.wneu.2016.03.031.
M. M. Grabowski et al., “Residual tumor volume versus extent of resection: Predictors of survival after surgery for glioblastoma,” J Neurosurg, vol. 121, no. 5, pp. 1115–1123, 2014, doi: 10.3171/2014.7.JNS132449.
A. M. Molinaro et al., “Association of Maximal Extent of Resection of Contrast-Enhanced and Non-Contrast-Enhanced Tumor with Survival Within Molecular Subgroups of Patients with Newly Diagnosed Glioblastoma,” JAMA Oncol, vol. 6, no. 4, pp. 495–503, 2020, doi: 10.1001/jamaoncol.2019.6143.
M. M. J. Wijnenga et al., “The impact of surgery in molecularly defined low-grade glioma: An integrated clinical, radiological, and molecular analysis,” Neuro Oncol, vol. 20, no. 1, pp. 103–112, 2018, doi: 10.1093/neuonc/nox176.
N. I. of H. (NIH), “External-beam radiation therapy.” https://www.cancer.gov/publications/dictionaries/cancer-terms/def/external-beam-radiation-therapy
F. Keime-Guibert et al., “Radiotherapy for Glioblastoma in the Elderly,” New England Journal of Medicine, vol. 356, no. 15, pp. 1527–1535, 2007, doi: 10.1056/nejmoa065901.
R. H. Press et al., “Optimal timing of chemoradiotherapy after surgical resection of glioblastoma: Stratification by validated prognostic classification,” Cancer, vol. 126, no. 14, pp. 3255–3264, 2020, doi: 10.1002/cncr.32797.
W. G. Breen et al., “Final report from Intergroup NCCTG 86-72-51 (Alliance): A phase III randomized clinical trial of high-dose versus low-dose radiation for adult low-grade glioma,” Neuro Oncol, vol. 22, no. 6, pp. 830–837, 2020, doi: 10.1093/neuonc/noaa021.
L. Khan, H. Soliman, A. Sahgal, J. Perry, W. Xu, and M. N. Tsao, “External beam radiation dose escalation for high grade glioma,” Cochrane Database of Systematic Reviews, vol. 2020, no. 5, 2020, doi: 10.1002/14651858.CD011475.pub3.
M. Niyazi et al., “ESTRO-ACROP guideline ‘target delineation of glioblastomas,’” Radiotherapy and Oncology, vol. 118, no. 1, pp. 35–42, 2016, doi: 10.1016/j.radonc.2015.12.003.
S. B. Nachbichler and F. W. Kreth, “Brachytherapy of Intracranial Gliomas,” Prog Neurol Surg, vol. 31, pp. 72–86, 2018, doi: 10.1159/000467114.
G. Minniti et al., “Radiotherapy plus concomitant and adjuvant temozolomide for glioblastoma in elderly patients,” J Neurooncol, vol. 88, no. 1, pp. 97–103, 2008, doi: 10.1007/s11060-008-9538-0.
M. M. Alonso, C. Gomez-Manzano, B. N. Bekele, W. K. A. Yung, and J. Fueyo, “Adenovirus-based strategies overcome temozolomide resistance by silencing the O6-methylguanine-DNA methyltransferase promoter,” Cancer Res, vol. 67, no. 24, pp. 11499–11504, 2007, doi: 10.1158/0008-5472.CAN-07-5312.
P. Zhang, Q. Xia, L. Liu, S. Li, and L. Dong, “Current Opinion on Molecular Characterization for GBM Classification in Guiding Clinical Diagnosis, Prognosis, and Therapy,” Front Mol Biosci, vol. 7, no. September, pp. 1–13, 2020, doi: 10.3389/fmolb.2020.562798.
M. Westphal et al., “A phase 3 trial of local chemotherapy with biodegradable carmustine (BCNU) wafers (Gliadel wafers) in patients with primary malignant glioma,” Neuro Oncol, vol. 5, no. 2, pp. 79–88, 2004, doi: 10.1215/s1522851702000236.
P. Y. Wen et al., “Glioblastoma in adults: A Society for Neuro-Oncology (SNO) and European Society of Neuro-Oncology (EANO) consensus review on current management and future directions,” Neuro Oncol, vol. 22, no. 8, pp. 1073–1113, 2020, doi: 10.1093/neuonc/noaa106.
A. J. Duplantier et al., “Mechanisms and therapeutic implications of hypermutation in gliomas,” Front Immunol, vol. 41, no. 1, pp. 139–148, 2021, doi: 10.1038/s41586-020-2209-9.Mechanisms.
K. L. Pitter et al., “Corticosteroids compromise survival in glioblastoma,” Brain, vol. 139, no. 5, pp. 1458–1471, 2016, doi: 10.1093/brain/aww046.
J. Sun, X. Gong, B. Purow, and Z. Zhao, “Uncovering MicroRNA and Transcription Factor Mediated Regulatory Networks in Glioblastoma,” PLoS Comput Biol, vol. 8, no. 7, p. e1002488, Jul. 2012, doi: 10.1371/journal.pcbi.1002488.
S. Srinivasan, I. R. P. Patric, and K. Somasundaram, “A Ten-microRNA Expression Signature Predicts Survival in Glioblastoma,” PLoS One, vol. 6, no. 3, p. e17438, Mar. 2011, doi: 10.1371/journal.pone.0017438.
J. Lu et al., “MicroRNA expression profiles classify human cancers,” Nature, vol. 435, no. 7043, pp. 834–838, Jun. 2005, doi: 10.1038/nature03702.
Y.-Y. Xu, P. Gao, Y. Sun, and Y.-R. Duan, “Development of targeted therapies in treatment of glioblastoma ,” Cancer Biol Med., vol. 12, no. 3, pp. 223–237, Sep. 2015.
A. B. Gaur, S. L. Holbeck, N. H. Colburn, and M. A. Israel, “Downregulation of Pdcd4 by mir-21 facilitates glioblastoma proliferation in vivo,” Neuro Oncol, vol. 13, no. 6, pp. 580–590, Jun. 2011, doi: 10.1093/neuonc/nor033.
P. Sathyan et al., “Mir-21–Sox2 Axis Delineates Glioblastoma Subtypes with Prognostic Impact,” The Journal of Neuroscience, vol. 35, no. 45, pp. 15097–15112, Nov. 2015, doi: 10.1523/JNEUROSCI.1265-15.2015.
M. S. Masoudi, E. Mehrabian, and H. Mirzaei, “MiR‐21: A key player in glioblastoma pathogenesis,” J Cell Biochem, vol. 119, no. 2, pp. 1285–1290, Feb. 2018, doi: 10.1002/jcb.26300.
S. K. Hermansen, B. S. Nielsen, C. Aaberg-Jessen, and B. W. Kristensen, “miR-21 Is Linked to Glioma Angiogenesis,” Journal of Histochemistry & Cytochemistry, vol. 64, no. 2, pp. 138–148, Feb. 2016, doi: 10.1369/0022155415623515.
S. Huang, N. Ali, L. Zhong, and J. Shi, “MicroRNAs as biomarkers for human glioblastoma: progress and potential,” Acta Pharmacol Sin, vol. 39, no. 9, pp. 1405–1413, Sep. 2018, doi: 10.1038/aps.2017.173.
A. Marisetty et al., “MiR-181 Family Modulates Osteopontin in Glioblastoma Multiforme,” Cancers (Basel), vol. 12, no. 12, p. 3813, Dec. 2020, doi: 10.3390/cancers12123813.
X. She et al., “miR-181 subunits enhance the chemosensitivity of temozolomide by Rap1B-mediated cytoskeleton remodeling in glioblastoma cells,” Medical Oncology, vol. 31, no. 4, p. 892, Apr. 2014, doi: 10.1007/s12032-014-0892-9.
H. Wang et al., “Upregulation of miR-181s reverses mesenchymal transition by targeting KPNA4 in glioblastoma,” Sci Rep, vol. 5, no. 1, p. 13072, Aug. 2015, doi: 10.1038/srep13072.
A. Marisetty et al., “MiR-181 Family Modulates Osteopontin in Glioblastoma Multiforme,” Cancers (Basel), vol. 12, no. 12, p. 3813, Dec. 2020, doi: 10.3390/cancers12123813.
J. Li et al., “Association Between Genetic Variant in the Promoter of Pri-miR-34b/c and Risk of Glioma,” Front Oncol, vol. 8, Sep. 2018, doi: 10.3389/fonc.2018.00413.
L. W. Macharia et al., “The genotypic and phenotypic impact of hypoxia microenvironment on glioblastoma cell lines,” BMC Cancer, vol. 21, no. 1, p. 1248, Nov. 2021, doi: 10.1186/s12885-021-08978-z.
G. Misso et al., “Mir-34: A New Weapon Against Cancer?,” Mol Ther Nucleic Acids, vol. 3, p. e195, Jan. 2014, doi: 10.1038/mtna.2014.47.
M. Yamakuchi and C. J. Lowenstein, “MiR-34, SIRT1, and p53: The feedback loop,” Cell Cycle, vol. 8, no. 5, pp. 712–715, Mar. 2009, doi: 10.4161/cc.8.5.7753.
M. A. Cortez et al., “PDL1 Regulation by p53 via miR-34,” JNCI: Journal of the National Cancer Institute, vol. 108, no. 1, Jan. 2016, doi: 10.1093/jnci/djv303.
N. Okada et al., “A positive feedback between p53 and miR-34 miRNAs mediates tumor suppression,” Genes Dev, vol. 28, no. 5, pp. 438–450, Mar. 2014, doi: 10.1101/gad.233585.113.
Z. Liu et al., “MiR-7-5p is frequently downregulated in glioblastoma microvasculature and inhibits vascular endothelial cell proliferation by targeting RAF1,” Tumor Biology, vol. 35, no. 10, pp. 10177–10184, Oct. 2014, doi: 10.1007/s13277-014-2318-x.
M. Chakrabarti and S. K. Ray, “Anti-tumor activities of luteolin and silibinin in glioblastoma cells: overexpression of miR-7-1-3p augmented luteolin and silibinin to inhibit autophagy and induce apoptosis in glioblastoma in vivo,” Apoptosis, vol. 21, no. 3, pp. 312–328, Mar. 2016, doi: 10.1007/s10495-015-1198-x.
Z. LIU et al., “miR-7 inhibits glioblastoma growth by simultaneously interfering with the PI3K/ATK and Raf/MEK/ERK pathways,” Int J Oncol, vol. 44, no. 5, pp. 1571–1580, May 2014, doi: 10.3892/ijo.2014.2322.
C. Yin, W. Kong, J. Jiang, H. Xu, and W. Zhao, “miR-7-5p inhibits cell migration and invasion in glioblastoma through targeting SATB1,” Oncol Lett, Nov. 2018, doi: 10.3892/ol.2018.9777.
C.-Z. Zhang et al., “MiR-221 and miR-222 target PUMA to induce cell survival in glioblastoma,” Mol Cancer, vol. 9, no. 1, p. 229, Dec. 2010, doi: 10.1186/1476-4598-9-229.
Kang, “miR-221/222 promote malignant progression of glioma through activation of the Akt pathway,” Int J Oncol, vol. 36, no. 4, Mar. 2010, doi: 10.3892/ijo_00000570.
C. Quintavalle et al., “miR-221/222 overexpession in human glioblastoma increases invasiveness by targeting the protein phosphate PTPμ,” Oncogene, vol. 31, no. 7, pp. 858–868, Feb. 2012, doi: 10.1038/onc.2011.280.
Z. Shi et al., “MiR-128 Inhibits Tumor Growth and Angiogenesis by Targeting p70S6K1,” PLoS One, vol. 7, no. 3, p. e32709, Mar. 2012, doi: 10.1371/journal.pone.0032709.
M. I. Almeida, R. M. Reis, and G. A. Calin, “MicroRNA history: Discovery, recent applications, and next frontiers,” Mutation Research/Fundamental and Molecular Mechanisms of Mutagenesis, vol. 717, no. 1–2, pp. 1–8, Dec. 2011, doi: 10.1016/j.mrfmmm.2011.03.009.
Q. Lei et al., “MicroRNA-based therapy for glioblastoma: Opportunities and challenges,” Eur J Pharmacol, vol. 938, p. 175388, Jan. 2023, doi: 10.1016/j.ejphar.2022.175388.
B. Banelli, A. Forlani, G. Allemanni, A. Morabito, M. P. Pistillo, and M. Romani, “MicroRNA in Glioblastoma: An Overview,” Int J Genomics, vol. 2017, pp. 1–16, 2017, doi: 10.1155/2017/7639084.
A. S. Pal and A. L. Kasinski, “Animal Models to Study MicroRNA Function,” 2017, pp. 53–118. doi: 10.1016/bs.acr.2017.06.006.
M. Ishida and F. M. Selaru, “miRNA-Based Therapeutic Strategies,” Curr Pathobiol Rep, vol. 1, no. 1, pp. 63–70, Mar. 2013, doi: 10.1007/s40139-012-0004-5.
J. Tabernero et al., “First-in-Humans Trial of an RNA Interference Therapeutic Targeting VEGF and KSP in Cancer Patients with Liver Involvement,” Cancer Discov, vol. 3, no. 4, pp. 406–417, Apr. 2013, doi: 10.1158/2159-8290.CD-12-0429.
M. Malhotra et al., “Targeted nanoparticle delivery of therapeutic antisense microRNAs presensitizes glioblastoma cells to lower effective doses of temozolomide in vitro and in a mouse model,” Oncotarget, vol. 9, no. 30, pp. 21478–21494, Apr. 2018, doi: 10.18632/oncotarget.25135.
P. Ofek et al., “Restoring the oncosuppressor activity of microRNA-34a in glioblastoma using a polyglycerol-based polyplex,” Nanomedicine, vol. 12, no. 7, pp. 2201–2214, Oct. 2016, doi: 10.1016/j.nano.2016.05.016.
R. D. Perrins et al., “Targeting Ultrasmall Gold Nanoparticles with cRGD Peptide Increases the Uptake and Efficacy of Cytotoxic Payload,” Nanomaterials, vol. 12, no. 22, p. 4013, Nov. 2022, doi: 10.3390/nano12224013.
K. K. Jain, “A Critical Overview of Targeted Therapies for Glioblastoma,” Front Oncol, vol. 8, Oct. 2018, doi: 10.3389/fonc.2018.00419.
E. Lozada-Delgado et al., “Targeting MicroRNA-143 Leads to Inhibition of Glioblastoma Tumor Progression,” Cancers (Basel), vol. 10, no. 10, p. 382, Oct. 2018, doi: 10.3390/cancers10100382.
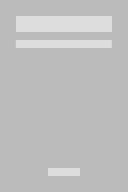
Opublikowane
Licencja

Utwór dostępny jest na licencji Creative Commons Uznanie autorstwa – Użycie niekomercyjne – Bez utworów zależnych 4.0 Międzynarodowe.