Fluorescencyjne wskaźniki aktywności neuronalnej i ich optymalizacja, jako narzędzie do analizy fizjologii mózgu
Słowa kluczowe:
fluorescencyjne wskaźniki, obrazowanie fluorescencyjne, GECI, GENI, farmakoterapiaStreszczenie
Neurobiolodzy nadal mają w dużym stopniu zawężoną wiedzę na temat wpływu zmian zachodzących w neuronach podczas przetwarzania informacji w mózgu zwierząt a tym bardziej ludzi. Aby poznać schemat, w jaki sposób sieci neuronowe są zdolne do kodowania oraz przechowywania danych trzeba zrozumieć proces pobierania przez nie informacji. Z tego względu konieczne jest śledzenie dynamicznych interakcji większych grup pojedynczych komórek w półkulach mózgu i nie tylko. Za pomocą metody obrazowania fluorescencyjnego jonów wapnia (Ca²⁺) możliwe jest analizowanie aktywności setek neuronów w mózgu między innymi u myszy, co umożliwia perspektywy badawcze całych sieci neuronowych. Dzięki genetycznie połączonym wskaźnikom Ca²⁺ neurony mogą być identyfikowane ze względu na ich genetyczny podtyp bądź połączenia. Rozwój w obrazowaniu przy użyciu fluorescencji umożliwił również obrazowanie neurotransmiterów i neuromodulatorów umożliwiając obrazowanie nie tylko aktywności neuronalnej ale i wpływu neuromodulatorów na konkretne receptory. Łącząc dostępne nowe technologie obrazowania neuronów oraz liczne badania, naukowcy mają szansę na poznanie schematu kodowania neuronowego w procesie przetwarzania bólu, dotyku oraz wielu innych istotnych sygnałów somatosensorycznych w mózgu człowieka. Poniższa praca ma na celu usystematyzowanie wiedzy na temat czujników fluorescencyjnych, drogi rozszerzania ich czułości, swoistości oraz jasności fluorescencji, która umożliwiła zastosowanie ich w badaniach nad mechanizmami mózgowymi. Podkreśla również istotę dalszego rozwijania metod obrazowania stosowanych w analizie bodźców neurochemicznych i farmakoterapii.
Bibliografia
Ramón y Cajal S. Histology of the Nervous System of Man and Vertebrates.; 1995.
Guillamón-Vivancos T, Vandael D, Torres D, López-Bendito G, Martini FJ. Mesoscale calcium imaging in vivo: evolution and contribution to developmental neuroscience. Front Neurosci. 2023;17:1210199.
Sherpa RT, Pala R, Mohieldin AM, Nauli SM. Measurement of cytoplasmic and cilioplasmic calcium in a single living cell. Methods Cell Biol. 2019;153:25-42.
Orrenius S, Zhivotovsky B, Nicotera P. Regulation of cell death: the calcium-apoptosis link. Nat Rev Mol Cell Biol. 2003;4(7):552-565.
Clapham DE. Calcium signaling. Cell. 2007;131(6):1047-1058.
McCombs JE, Palmer AE. Measuring calcium dynamics in living cells with genetically encodable calcium indicators. Methods. 2008;46(3):152-159.
Toth AB, Shum AK, Prakriya M. Regulation of neurogenesis by calcium signaling. Cell Calcium. 2016;59(2-3):124-134.
Arjun McKinney A, Petrova R, Panagiotakos G. Calcium and activity-dependent signaling in the developing cerebral cortex. Development. 2022;149(17). doi:10.1242/dev.198853
Grienberger C, Konnerth A. Imaging calcium in neurons. Neuron. 2012;73(5):862-885.
Berridge MJ, Bootman MD, Roderick HL. Calcium signalling: dynamics, homeostasis and remodelling. Nat Rev Mol Cell Biol. 2003;4(7):517-529.
Ashley CC, Ridgway EB. Simultaneous Recording of Membrane Potential, Calcium Transient and Tension in Single Muscle Fibres. Nature. 1968;219(5159):1168-1169.
Ashley CC, Ridgway EB. On the relationships between membrane potential, calcium transient and tension in single barnacle muscle fibres. J Physiol. 1970;209(1):105-130.
Tsien RY. Fluorescent probes of cell signaling. Annu Rev Neurosci. 1989;12:227-253.
Hotez PJ, Bottazzi ME, Franco-Paredes C, Ault SK, Periago MR. The Neglected Tropical Diseases of Latin America and the Caribbean: A Review of Disease Burden and Distribution and a Roadmap for Control and Elimination. PLoS Negl Trop Dis. 2008;2(9):e300.
Dana H, Mohar B, Sun Y, et al. Sensitive red protein calcium indicators for imaging neural activity. Elife. 2016;5. doi:10.7554/eLife.12727
Kim SA, Tai CY, Mok LP, Mosser EA, Schuman EM. Calcium-dependent dynamics of cadherin interactions at cell-cell junctions. Proc Natl Acad Sci U S A. 2011;108(24):9857-9862.
Akerboom J, Chen TW, Wardill TJ, et al. Optimization of a GCaMP calcium indicator for neural activity imaging. J Neurosci. 2012;32(40):13819-13840.
Tian L, Hires SA, Mao T, et al. Imaging neural activity in worms, flies and mice with improved GCaMP calcium indicators. Nat Methods. 2009;6(12):875-881.
Palmer AE, Tsien RY. Measuring calcium signaling using genetically targetable fluorescent indicators. Nat Protoc. 2006;1(3):1057-1065.
Miyawaki A, Llopis J, Heim R, et al. Fluorescent indicators for Ca2+ based on green fluorescent proteins and calmodulin. Nature. 1997;388(6645):882-887.
Zhang Y, Rózsa M, Liang Y, et al. Fast and sensitive GCaMP calcium indicators for imaging neural populations. Nature. 2023;615(7954):884-891.
Baird GS, Zacharias DA, Tsien RY. Circular permutation and receptor insertion within green fluorescent proteins. Proc Natl Acad Sci U S A. 1999;96(20):11241-11246.
Dana H, Sun Y, Mohar B, et al. High-performance calcium sensors for imaging activity in neuronal populations and microcompartments. Nat Methods. 2019;16(7):649-657.
Inoue M, Takeuchi A, Manita S, et al. Rational Engineering of XCaMPs, a Multicolor GECI Suite for In Vivo Imaging of Complex Brain Circuit Dynamics. Cell. 2019;177(5):1346-1360.e24.
Chen TW, Wardill TJ, Sun Y, et al. Ultrasensitive fluorescent proteins for imaging neuronal activity. Nature. 2013;499(7458):295-300.
Shcherbakova DM, Shemetov AA, Kaberniuk AA, Verkhusha VV. Natural photoreceptors as a source of fluorescent proteins, biosensors, and optogenetic tools. Annu Rev Biochem. 2015;84:519-550.
Shemetov AA, Monakhov MV, Zhang Q, et al. A near-infrared genetically encoded calcium indicator for in vivo imaging. Nat Biotechnol. 2021;39(3):368-377.
Knöpfel T, Song C. Optical voltage imaging in neurons: moving from technology development to practical tool. Nat Rev Neurosci. 2019;20(12):719-727.
Panzera LC, Hoppa MB. Genetically Encoded Voltage Indicators Are Illuminating Subcellular Physiology of the Axon. Front Cell Neurosci. 2019;13:52.
Pal A, Tian L. Imaging voltage and brain chemistry with genetically encoded sensors and modulators. Curr Opin Chem Biol. 2020;57:166-176.
Guillaumin MCC, Burdakov D. Neuropeptides as Primary Mediators of Brain Circuit Connectivity. Front Neurosci. 2021;15:644313.
Kintos N, Nusbaum MP, Nadim F. Convergent neuromodulation onto a network neuron can have divergent effects at the network level. J Comput Neurosci. 2016;40(2):113-135.
Sabatini BL, Tian L. Imaging Neurotransmitter and Neuromodulator Dynamics In Vivo with Genetically Encoded Indicators. Neuron. 2020;108(1):17-32.
Helassa N, Dürst CD, Coates C, et al. Ultrafast glutamate sensors resolve high-frequency release at Schaffer collateral synapses. Proc Natl Acad Sci U S A. 2018;115(21):5594-5599.
Shivange AV, Borden PM, Muthusamy AK, et al. Determining the pharmacokinetics of nicotinic drugs in the endoplasmic reticulum using biosensors. J Gen Physiol. 2019;151(6):738-757.
Marvin JS, Shimoda Y, Magloire V, et al. A genetically encoded fluorescent sensor for in vivo imaging of GABA. Nat Methods. 2019;16(8):763-770.
Lobas MA, Tao R, Nagai J, et al. A genetically encoded single-wavelength sensor for imaging cytosolic and cell surface ATP. Nat Commun. 2019;10(1):711.
Keller JP, Marvin JS, Lacin H, et al. In vivo glucose imaging in multiple model organisms with an engineered single-wavelength sensor. Cell Rep. 2021;35(12):109284.
Mita M, Ito M, Harada K, et al. Green Fluorescent Protein-Based Glucose Indicators Report Glucose Dynamics in Living Cells. Anal Chem. 2019;91(7):4821-4830.
Unger EK, Keller JP, Altermatt M, et al. Directed Evolution of a Selective and Sensitive Serotonin Sensor via Machine Learning. Cell. 2020;183(7):1986-2002.e26.
Wang H, Jing M, Li Y. Lighting up the brain: genetically encoded fluorescent sensors for imaging neurotransmitters and neuromodulators. Curr Opin Neurobiol. 2018;50:171-178.
Patriarchi T, Cho JR, Merten K, et al. Ultrafast neuronal imaging of dopamine dynamics with designed genetically encoded sensors. Science. 2018;360(6396). doi:10.1126/science.aat4422
Sun F, Zeng J, Jing M, et al. A Genetically Encoded Fluorescent Sensor Enables Rapid and Specific Detection of Dopamine in Flies, Fish, and Mice. Cell. 2018;174(2):481-496.e19.
Jing M, Li Y, Zeng J, et al. An optimized acetylcholine sensor for monitoring in vivo cholinergic activity. Nat Methods. 2020;17(11):1139-1146.
Feng J, Zhang C, Lischinsky JE, et al. A Genetically Encoded Fluorescent Sensor for Rapid and Specific In Vivo Detection of Norepinephrine. Neuron. 2019;102(4):745-761.e8.
Dong C, Ly C, Dunlap LE, et al. Psychedelic-inspired drug discovery using an engineered biosensor. Cell. 2021;184(10):2779-2792.e18.
Peng W, Wu Z, Song K, Zhang S, Li Y, Xu M. Regulation of sleep homeostasis mediator adenosine by basal forebrain glutamatergic neurons. Science. 2020;369(6508). doi:10.1126/science.abb0556
Wu Z, He K, Chen Y, et al. A sensitive GRAB sensor for detecting extracellular ATP in vitro and in vivo. Neuron. 2022;110(5):770-782.e5.
Dong A, He K, Dudok B, et al. A fluorescent sensor for spatiotemporally resolved imaging of endocannabinoid dynamics in vivo. Nat Biotechnol. 2022;40(5):787-798.
Marvin JS, Scholl B, Wilson DE, et al. Stability, affinity, and chromatic variants of the glutamate sensor iGluSnFR. Nat Methods. 2018;15(11):936-939.
Marvin JS, Schreiter ER, Echevarría IM, Looger LL. A genetically encoded, high-signal-to-noise maltose sensor. Proteins. 2011;79(11):3025-3036.
Pachitariu M, Stringer C, Dipoppa M, et al. Suite2p: beyond 10,000 neurons with standard two-photon microscopy. bioRxiv. Published online June 30, 2016. doi:10.1101/061507
Sanchez-Tecuatl M, Vargaz-Guadarrama A, Ramirez-Cortes JM, Gomez-Gil P, Moccia F, Berra-Romani R. Automated Intracellular Calcium Profiles Extraction from Endothelial Cells Using Digital Fluorescence Images. Int J Mol Sci. 2018;19(11). doi:10.3390/ijms19113440
Giovannucci A, Friedrich J, Gunn P, et al. CaImAn an open source tool for scalable calcium imaging data analysis. Elife. 2019;8. doi:10.7554/eLife.38173
Gauthier JL, Tank DW. A Dedicated Population for Reward Coding in the Hippocampus. Neuron. 2018;99(1):179-193.e7.
Harris KD, Quiroga RQ, Freeman J, Smith SL. Improving data quality in neuronal population recordings. Nat Neurosci. 2016;19(9):1165-1174.
Lecoq J, Savall J, Vučinić D, et al. Visualizing mammalian brain area interactions by dual-axis two-photon calcium imaging. Nat Neurosci. 2014;17(12):1825-1829.
Gauthier JL, Koay SA, Nieh EH, Tank DW, Pillow JW, Charles AS. Detecting and correcting false transients in calcium imaging. Nat Methods. 2022;19(4):470-478.
May PJ. The mammalian superior colliculus: laminar structure and connections. Prog Brain Res. 2006;151:321-378.
Feinberg EH, Meister M. Orientation columns in the mouse superior colliculus. Nature. 2015;519(7542):229-232.
Savier EL, Chen H, Cang J. Effects of Locomotion on Visual Responses in the Mouse Superior Colliculus. J Neurosci. 2019;39(47):9360-9368.
Li YT, Turan Z, Meister M. Functional Architecture of Motion Direction in the Mouse Superior Colliculus. Curr Biol. 2020;30(17):3304-3315.e4.
Li Z, Wu R, Li YT. Calcium Imaging in Mouse Superior Colliculus. J Vis Exp. 2023;(194). doi:10.3791/65181
Wessels WJ, Marani E. A rostrocaudal somatotopic organization in the brachial dorsal root ganglia of neonatal rats. Clin Neurol Neurosurg. 1993;95 Suppl:S3-S11.
Sørensen B, Tandrup T, Koltzenburg M, Jakobsen J. No further loss of dorsal root ganglion cells after axotomy in p75 neurotrophin receptor knockout mice. J Comp Neurol. 2003;459(3):242-250.
Liu Y, Ma Q. Generation of somatic sensory neuron diversity and implications on sensory coding. Curr Opin Neurobiol. 2011;21(1):52-60.
Stucky CL, Mikesell AR. Cutaneous pain in disorders affecting peripheral nerves. Neurosci Lett. 2021;765:136233.
Kucharczyk MW, Chisholm KI, Denk F, Dickenson AH, Bannister K, McMahon SB. The impact of bone cancer on the peripheral encoding of mechanical pressure stimuli. Pain. 2020;161(8):1894-1905.
MacDonald DI, Luiz AP, Iseppon F, Millet Q, Emery EC, Wood JN. Silent cold-sensing neurons contribute to cold allodynia in neuropathic pain. Brain. 2021;144(6):1711-1726.
Wang F, Bélanger E, Côté SL, et al. Sensory Afferents Use Different Coding Strategies for Heat and Cold. Cell Rep. 2018;23(7):2001-2013.
Iseppon F, Linley JE, Wood JN. Calcium imaging for analgesic drug discovery. Neurobiol Pain. 2022;11:100083.
Kim YS, Chu Y, Han L, et al. Central terminal sensitization of TRPV1 by descending serotonergic facilitation modulates chronic pain. Neuron. 2014;81(4):873-887.
Shannonhouse J, Gomez R, Son H, Zhang Y, Kim YS. In Vivo Calcium Imaging of Neuronal Ensembles in Networks of Primary Sensory Neurons in Intact Dorsal Root Ganglia. J Vis Exp. 2023;(192). doi:10.3791/64826
Kovács GL. The Endocrine Brain: Pathophysiological Role of Neuropeptide-Neurotransmitter Interactions. EJIFCC. 2004;15(3):107-112.
Hu H, Wei Y, Wang D, et al. Glucose monitoring in living cells with single fluorescent protein-based sensors. RSC Adv. 2018;8(5):2485-2489.
Nadim F, Bucher D. Neuromodulation of neurons and synapses. Curr Opin Neurobiol. 2014;29:48-56.
Hauser AS, Attwood MM, Rask-Andersen M, Schiöth HB, Gloriam DE. Trends in GPCR drug discovery: new agents, targets and indications. Nat Rev Drug Discov. 2017;16(12):829-842.
Shoichet BK, Kobilka BK. Structure-based drug screening for G-protein-coupled receptors. Trends Pharmacol Sci. 2012;33(5):268-272.
Smith JS, Lefkowitz RJ, Rajagopal S. Biased signalling: from simple switches to allosteric microprocessors. Nat Rev Drug Discov. 2018;17(4):243-260.
Whalen EJ, Rajagopal S, Lefkowitz RJ. Therapeutic potential of β-arrestin- and G protein-biased agonists. Trends Mol Med. 2011;17(3):126-139.
Chi T, Gold JA. A review of emerging therapeutic potential of psychedelic drugs in the treatment of psychiatric illnesses. J Neurol Sci. 2020;411:116715.
Cameron LP, Tombari RJ, Lu J, et al. A non-hallucinogenic psychedelic analogue with therapeutic potential. Nature. 2021;589(7842):474-479.
Dong C, Zheng Y, Long-Iyer K, Wright EC, Li Y, Tian L. Fluorescence Imaging of Neural Activity, Neurochemical Dynamics, and Drug-Specific Receptor Conformation with Genetically Encoded Sensors. Annu Rev Neurosci. 2022;45:273-294.
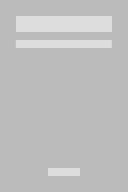
Opublikowane
Licencja

Utwór dostępny jest na licencji Creative Commons Uznanie autorstwa – Użycie niekomercyjne – Bez utworów zależnych 4.0 Międzynarodowe.